Research Activities of Our Group
Our group conducts theoretical research on electronic states in solids from the perspective of exploring novel properties arising from electronic correlations. Condensed matter physics, which explores Avogadro-scale ensembles of electrons, is particularly attractive as it addresses the diverse phenomena arising from the complex interplay between quantum mechanics and many-body effects driven by electron-electron interactions. While electrons are elementary particles, their collective behavior can create nontrivial ground states. When the ground state is viewed as a vacuum, excitations from this state are called "quasiparticles." If quantum many-body effects lead to the realization of a nontrivial ground state, the quasiparticles emerging from it may possess entirely different properties from the original electrons. Such quasiparticles may exhibit intriguing characteristics as emergent quasiparticles, such as Majorana fermions or anyons. Conversely, exploring ground states that give rise to such novel quasiparticles with unique features and functionalities is also one of the interests of research. This challenging theme, akin to designing a vacuum, is a major target of our group, which primarily investigates strongly correlated electron systems.Recent Research Topics
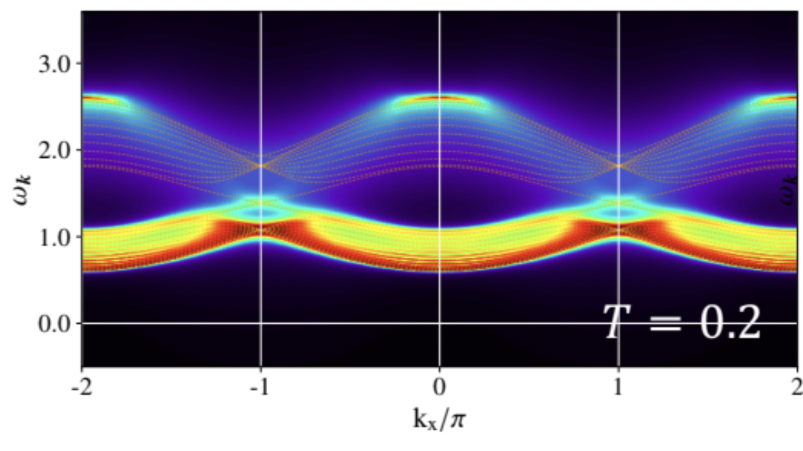
This study constructed a theoretical framework for analyzing elementary excitations in localized quantum models that describe interactions among multiple localized degrees of freedom at finite temperatures.
In previous studies, elementary excitations in general localized electronic models were explored using linear flavor-wave theory, which is a generalization of linear spin-wave theory to SU(N).
However, in cases where quantum fluctuations are significant, nonlinear terms describing interactions between quasiparticles are known to play a crucial role.
We extended the linear flavor-wave theory to a nonlinear formulation, developing a theory that appropriately addresses quasiparticle interactions at finite temperatures.
Applying this method to the Kitaev model under a magnetic field, which exhibits topological magnons, we revealed that in the weak magnetic field region, damping due to interactions among magnon edge modes becomes significant, and the damping rate increases with rising temperature.
Since our approach starts from a general localized effective Hamiltonian, it is widely applicable to localized electronic systems derived from multi-orbital Hubbard models in the strongly correlated limit.
S. Koyama and J. Nasu: Phys. Rev. B 108, 235162 (2023).
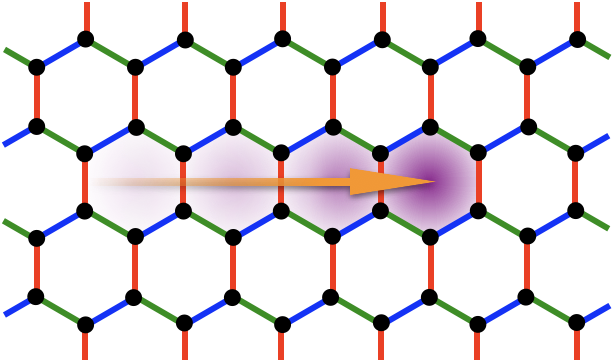
In the Kitaev quantum spin liquid, quantum spins behave as if split into itinerant Majorana fermions and localized excitations called visons.
Under a magnetic field, the Majorana fermion system forms topologically nontrivial bands, and the vison excitation traps Majorana particles.
The Majorana fermions localized at the vison act as Majorana zero modes, and this composite particle behaves as a non-Abelian anyon.
Since non-Abelian anyons are expected to serve as robust quantum computational elements in topological quantum computation, the observation, generation, and control of visons are critical challenges.
However, even in the ideal Kitaev model, real-time control methods remain largely unexplored.
In this study, we developed a theory for the real-time control of vison excitations in Kitaev quantum spin liquids using magnetic fields.
C. Harada, A. Ono, and J. Nasu: Phys. Rev. B 108, L241118 (2023).
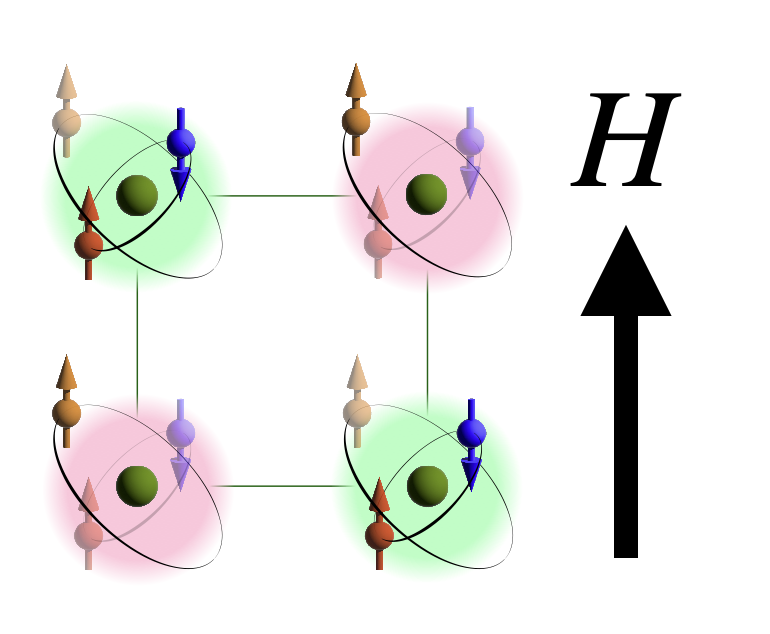
In perovskite cobalt oxides, cobalt ions exhibit multiple spin states (low spin, intermediate spin, high spin) due to the competition between crystal fields and Hund's coupling, significantly affecting their magnetic properties.
Quantum mechanical mixing of different spin states in this competitive region is expected, interpreted as excitonic condensation.
Since this competition can be induced by magnetic fields, we comprehensively investigated the magnetic field effects of a two-orbital Hubbard model using Hartree-Fock approximation and mean-field approximation for strongly correlated effective models.
As a result, we found that two different types of excitonic condensation states can be realized under magnetic fields.
One of these involves spin-state order and can be interpreted as an excitonic supersolid state.
Experimental results suggest the induction of multiple phases under ultra-strong magnetic fields in cobalt oxides, and our theoretical study corresponds to the possibility of excitonic condensation and excitonic supersolid states.
T. Tatsuno, E. Mizoguchi, J. Nasu, M. Naka, S. Ishihara: J. Phys. Soc. Jpn. 85, 083706 (2016).
M. Naka, E. Mizoguchi, J. Nasu, and S. Ishihara: J. Phys. Soc. Jpn. 87, 063709 (2018).
A. Ikeda, Y. H. Matsuda, K. Sato, Y. Ishii, H. Sawabe, D. Nakamura, S. Takeyama, and J. Nasu: Nat. Commun.
14, 1744 (2023).
R. Koga and J. Nasu: J. Phys. Soc. Jpn. 93, 054703 (2024).
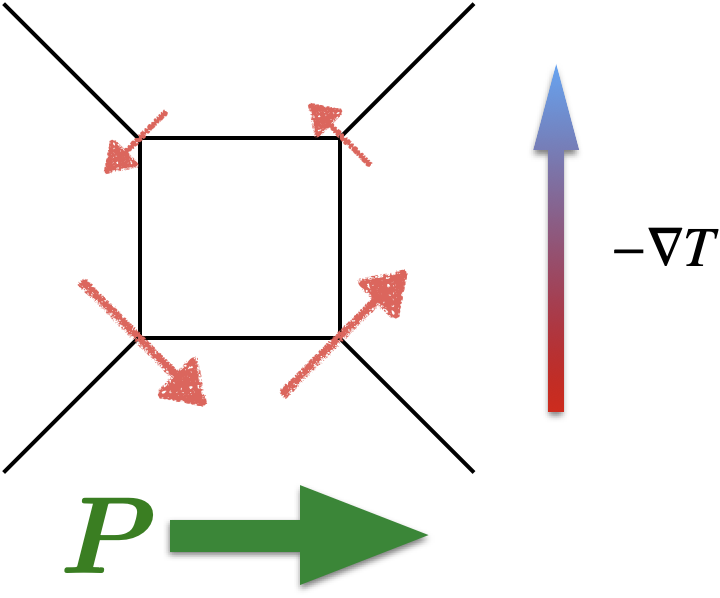
In strongly correlated electron systems, various ordered states emerge due to electron-electron interactions, resulting in unique response phenomena.
These ordered states include charge order, magnetic order, and orbital order, often categorized using multipoles.
Recently, discussions have arisen about toroidal moments that differ from magnetization and electric polarization under time and spatial inversion symmetry.
These include electric and magnetic toroidal multipoles, contributing to intriguing response phenomena and transport properties.
While electric toroidal dipole order has been less discussed due to the scarcity of candidate materials, this study introduces a spinless three-orbital model as the simplest model for electric toroidal dipole order.
By analyzing ordered states in the strongly correlated regime, we particularly focused on thermal response properties and used an extended linear response theory to analyze the electric polarization response to temperature gradients.
The results revealed that in parameter regions where electric toroidal dipole order arises, electric polarization appears perpendicular to the applied temperature gradient.
The response tensor in this case consists solely of antisymmetric components, attributed to the electric toroidal dipole order.
J. Nasu and S. Hayami: Phys. Rev. B 105, 245125 (2022).
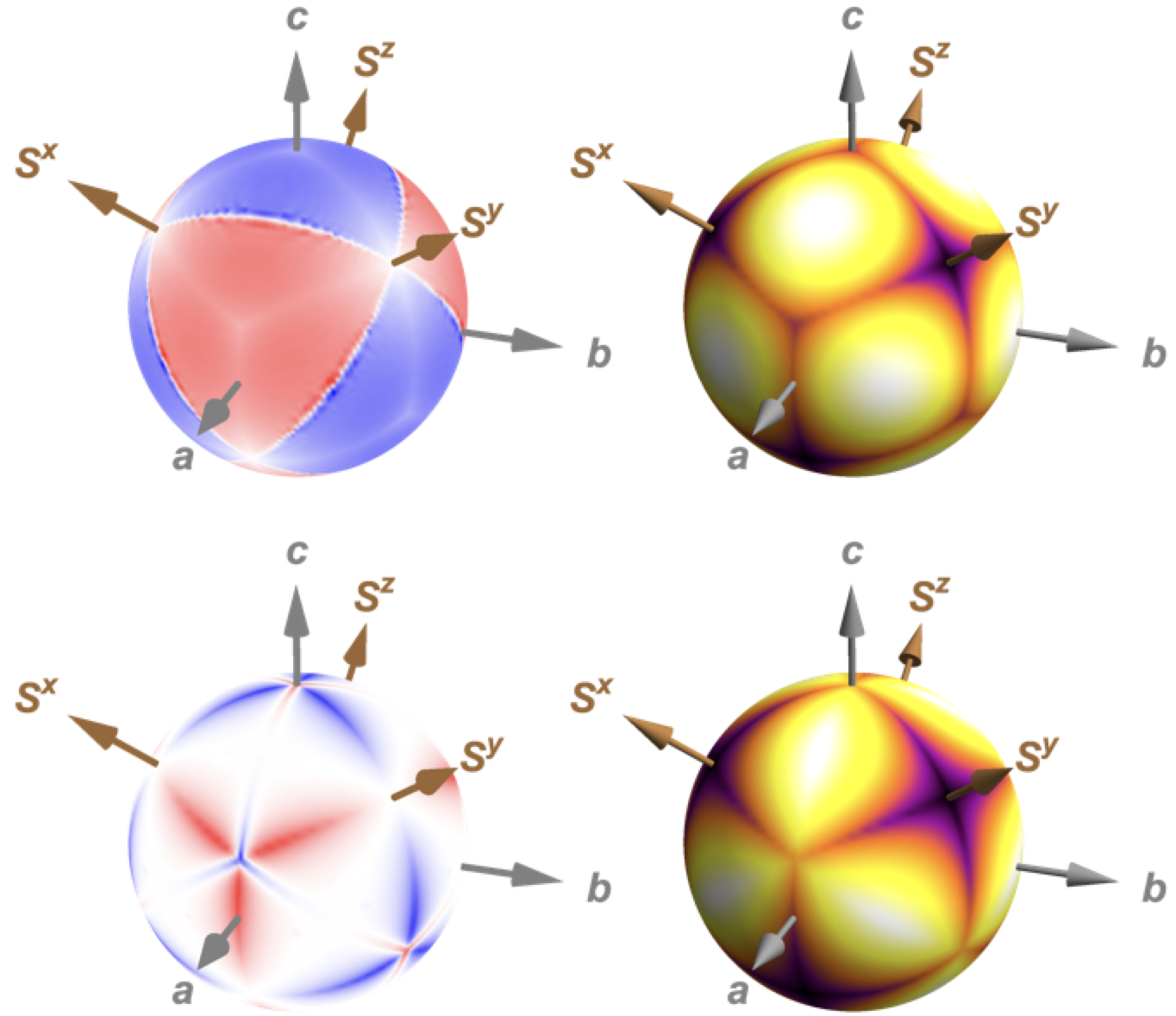
The thermal transport phenomena of quantum spin models based on the Kitaev model have recently attracted considerable attention.
This study examined the magnetic field-angle dependence of thermal Hall conductivity, assuming magnons as heat carriers.
The results showed that for magnons, the excitation gap does not disappear when the sign of the thermal Hall conductivity reverses, in contrast to the case of Majorana particles.
Additionally, when the spin configuration is non-coplanar, the thermal Hall conductivity increases.
Comprehensive analysis of the thermal Hall conductivity with changing magnetic field directions provided new insights into the role of off-diagonal interactions.
S. Koyama and J. Nasu: Phys. Rev. B 104, 075121-1-13 (2021).
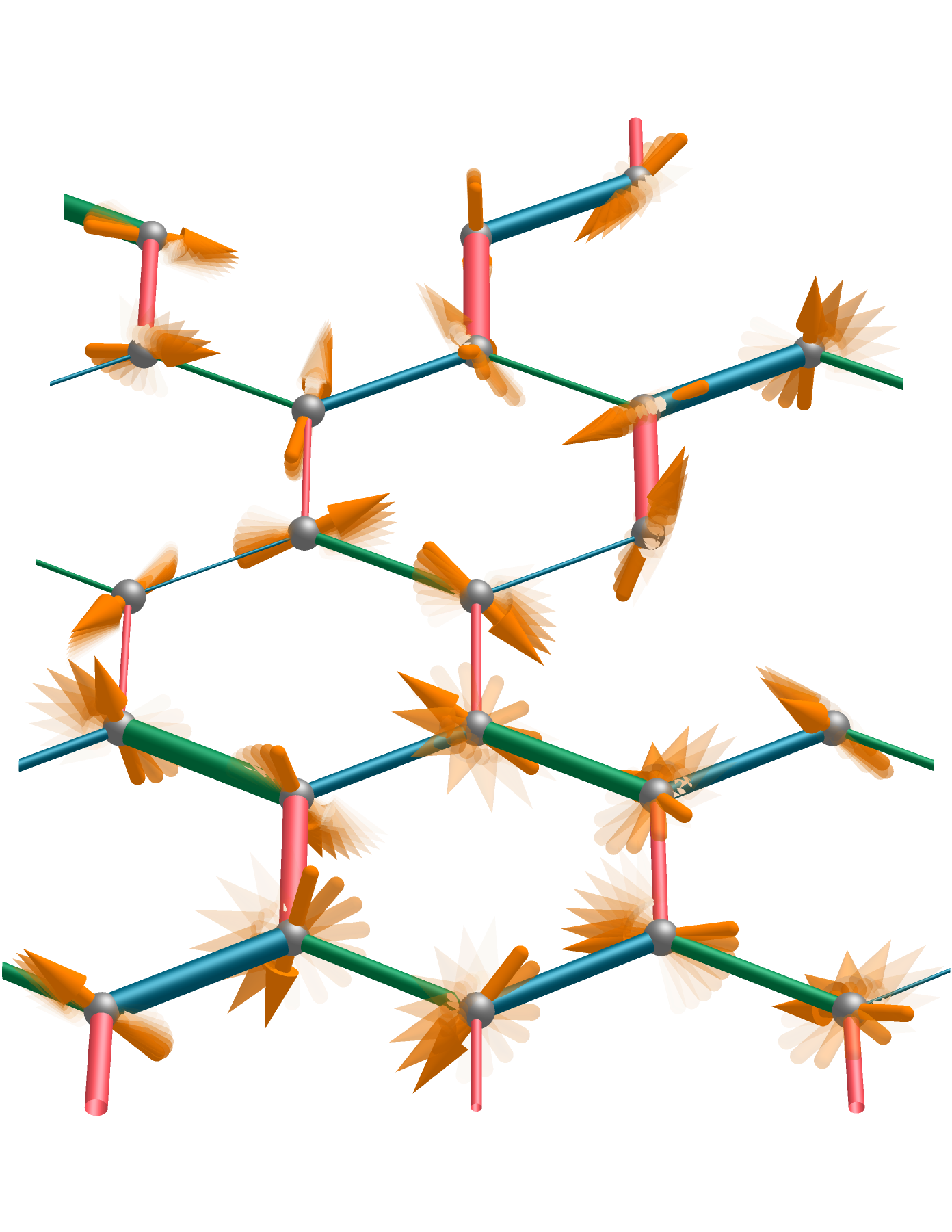
A novel iridium oxide material, which remains magnetically disordered down to extremely low temperatures, has been synthesized recently.
The impact of disorder effects caused by hydrogen position uncertainty, in addition to Kitaev interactions, has been pointed out in the realization of this disordered state.
Motivated by this, we used quantum Monte Carlo simulations to investigate the effects of disorder on the magnetic dynamics of the Kitaev quantum spin liquid.
We considered two types of randomness: site defects and interaction randomness, and explored their differences.
The results revealed distinct behaviors in specific heat temperature dependence, thermal transport properties, and magnetic excitation spectra for the two types of disorder.
These findings provide valuable insights into identifying the dominant type of disorder in real materials.
J. Nasu and Y. Motome: Phys. Rev. B 102, 054437 (2020).
J. Nasu and Y. Motome: Phys. Rev. B 104, 035116 (2021).
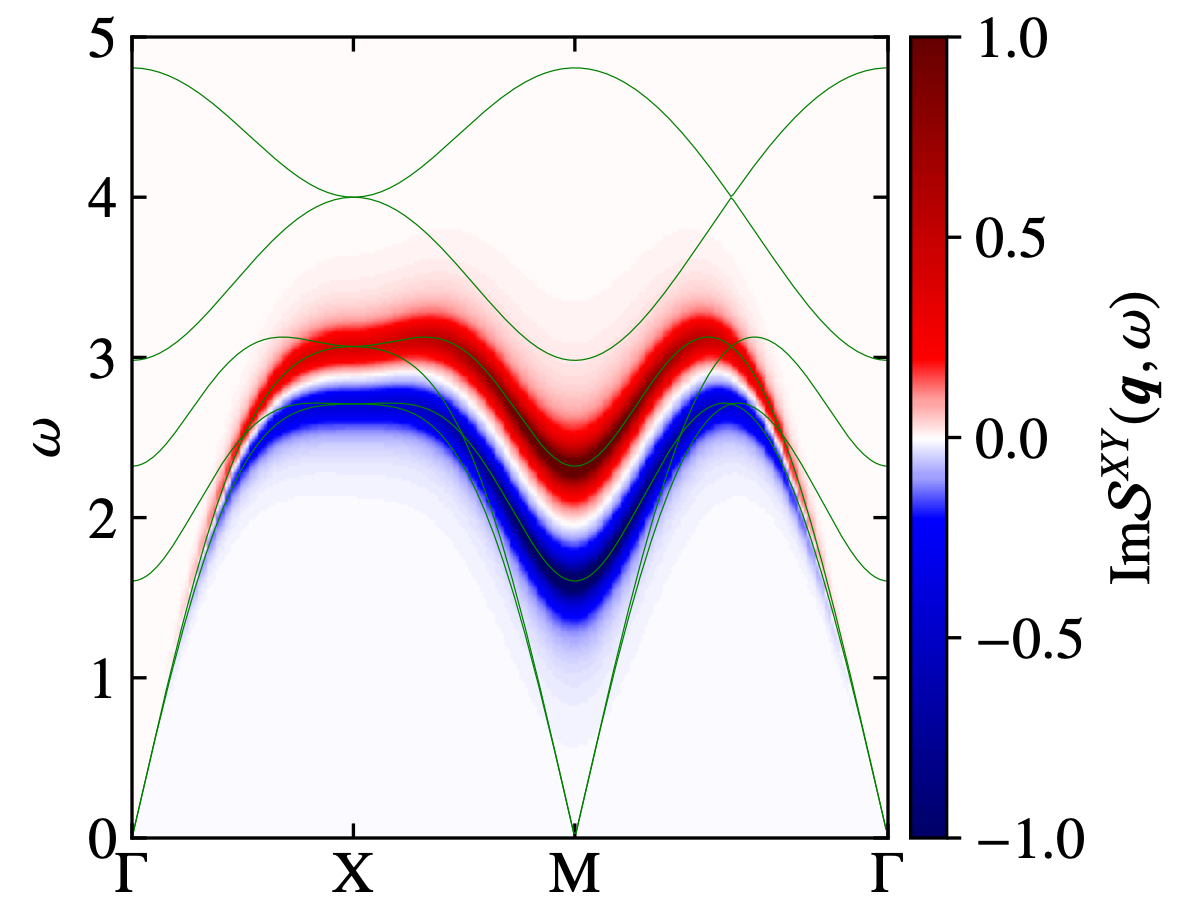
Cobalt oxides have attracted attention as candidate materials for excitonic insulators.
In these materials, the excitonic insulating state is thought to be realized through the quantum mechanical superposition of different spin states.
Despite breaking time-reversal symmetry, this state lacks magnetization, making its experimental identification challenging.
This study started with a two-orbital Hubbard model and applied spin-wave theory to an effective model derived through perturbative expansion from the strongly correlated limit.
The results revealed that the excitonic insulating state induces spin splitting in magnetic excitation spectra, even in the absence of magnetization.
Furthermore, this spin splitting leads to the spin Seebeck effect.
These findings suggest a novel method for identifying excitonic condensation, which have been difficult to detect until now.
J. Nasu and M. Naka: Phys. Rev. B 103, L121104 (2021).
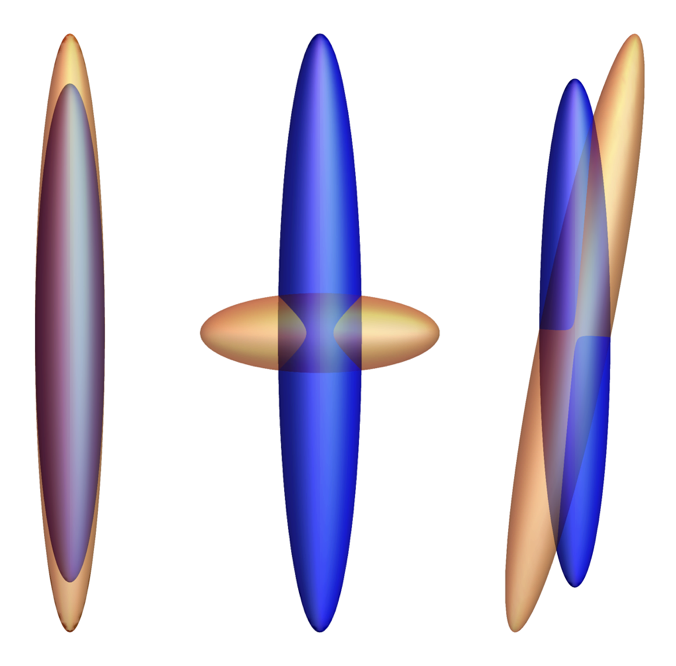
Cobalt oxides, studied extensively as Mott insulators with spin-state degrees of freedom, have attracted attention as candidate materials for excitonic insulators.
Due to the incomplete quenching of orbital angular momentum and the significant role of spin-orbit interactions, incorporating spin-orbit interactions is necessary to understand excitonic condensation phases in cobalt oxides.
This study aimed to clarify the magnetic field effects in strongly correlated electron systems with spin-state degrees of freedom, incorporating spin-orbit interactions into an effective model derived from a two-orbital Hubbard model.
The analysis revealed a significant enhancement of magnetic susceptibility near the phase boundary between low-spin states and excitonic insulator states under a magnetic field.
This peculiar behavior was attributed to spin nematic fluctuations inherent to high-spin states stabilized by Hund's coupling.
These findings not only elucidate the influence of spin fluctuations on excitonic condensation but also highlight the importance of quantum spin nematic fluctuations in this state.
J. Nasu, M. Naka, S. Ishihara: Phys. Rev. B 102, 045143 (2020).
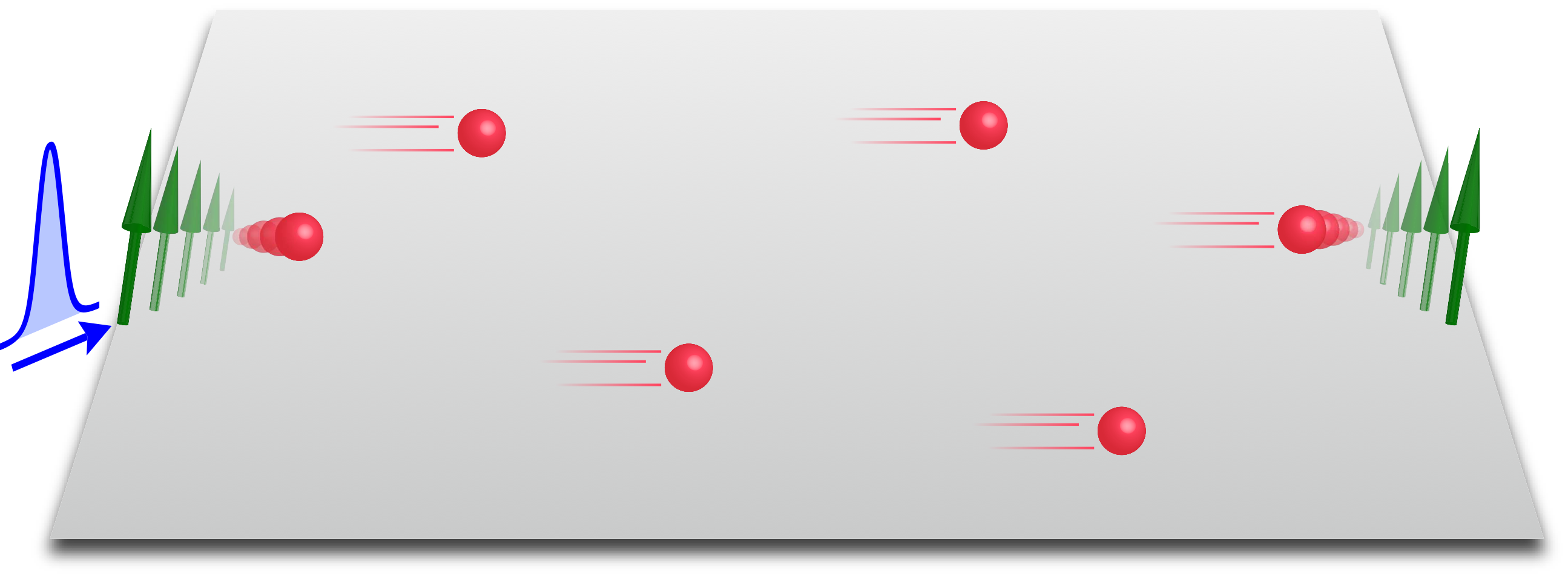
In typical magnetic insulators, the motion of electron spins, the origin of magnetism, propagates as waves via magnetic interactions within the crystal.
In contrast, in Kitaev quantum spin liquids, spin modulations excited by pulsed magnetic fields applied at one edge rapidly decay without propagating through the material, leaving the electron spins inside the material unchanged over time.
However, this study discovered that, after a certain period, the electron spins at the right edge suddenly begin to move.
In the Kitaev spin liquid, electron spins appear to behave as if they are split into two types of Majorana particles.
While one type is localized, the other is itinerant.
The analysis showed that the time evolution of electron spins excited by pulsed magnetic fields quickly converts into itinerant Majorana fermions, propagating in the material without causing spin modulations, but inducing time evolution of electron spins at the right edge.
These results suggest that spin excitations are mediated by fractional quasiparticles, in particular, Majorana fermions.
T. Minakawa, Y. Murakami, A. Koga, and J. Nasu: Phys. Rev. Lett. 125, 047204 (2020).
A. Koga, T. Minakawa, Y. Murakami, and J. Nasu: J. Phys. Soc. Jpn. 89, 033701 (2020).
H. Taguchi, Y. Murakami, A. Koga, and J. Nasu: Phys. Rev. B 104, 125139 (2021).
J. Nasu, Y. Murakami, and A. Koga: Phys. Rev. B 106, 024411 (2022).
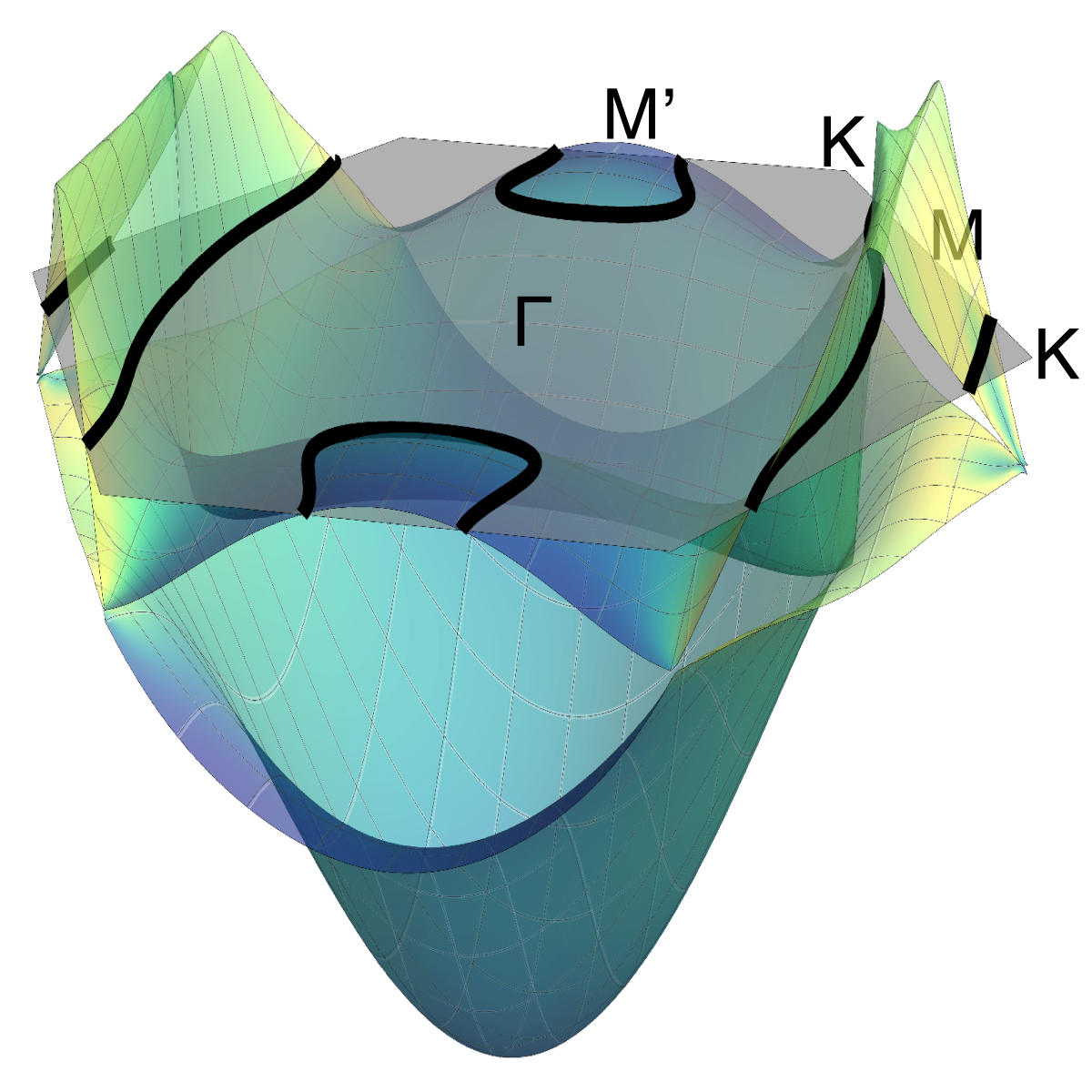
Quantum spin liquids, which lack explicit order parameters, have been under debate regarding their characterization.
Recent experiments have focused on the fractionalization of spins in quantum spin liquids, such as asymptotic behavior of specific heat at extremely low temperatures.
However, theoretical calculations at finite temperatures are essential for comparison with these experimental results, though understanding quantum spin liquid properties is challenging even at absolute zero.
To capture the prominent feature of quantum spin fractionalization, we conducted a theoretical study focusing on the non-equilibrium dynamics of the Kitaev model, a system that rigorously exhibits a quantum spin liquid ground state.
By extending the Majorana mean-field theory to real-time dynamics, we computed transient spin dynamics under sudden magnetic field changes.
The dynamical spin structure factor obtained from minor magnetic field quenching qualitatively matched exact results calculated via the Kubo formula.
Furthermore, for the intermediate phase induced by magnetic fields in the antiferromagnetic Kitaev interaction case, the transient spectra after a sudden zeroing of the magnetic field revealed that high-energy structures reflecting Majorana quasiparticles rapidly disappear.
Instead, oscillations on the flux energy scale persist for an extended duration.
This result indicates that excitations with distinct energy scales manifest on separate timescales in real-time dynamics, offering observable phenomena linked to two types of quasiparticle excitations.
J. Nasu, and Y. Motome: Phys. Rev. Research 1, 033007 (2019).
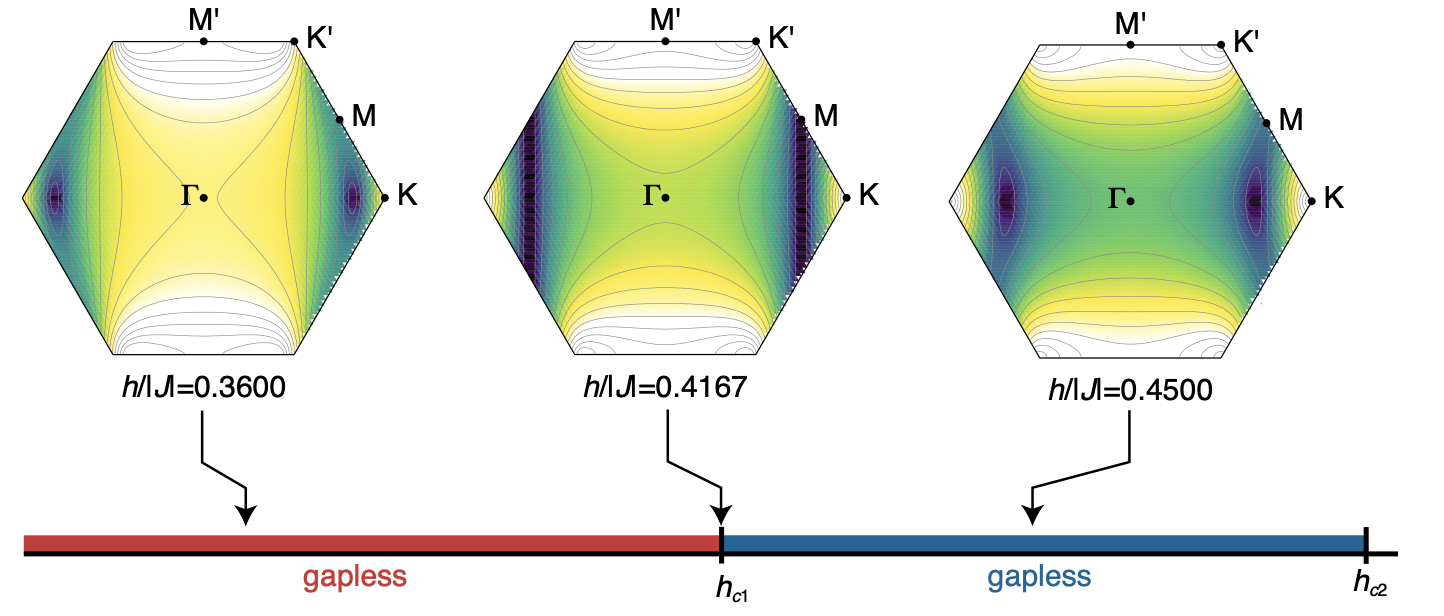
This study investigated quantum phase transitions in the Kitaev model under a magnetic field, focusing on the topological properties of fractional quasiparticles called Majorana excitations.
Specifically, we applied Majorana mean-field theory to the antiferromagnetic Kitaev model under a magnetic field and discovered a novel gapless phase between the low-field gapless quantum spin liquid and the high-field forced ferromagnetic state.
The phase transition between the two gapless phases is driven by topological changes in the bands formed by Majorana quasiparticles.
These peculiar topological changes were found to be justified by a sign change in the effective Kitaev interaction induced by the magnetic field.
J. Nasu, Y. Kato, Y. Kamiya, and Y. Motome: Phys. Rev. B 98, 060416(R) (2018).
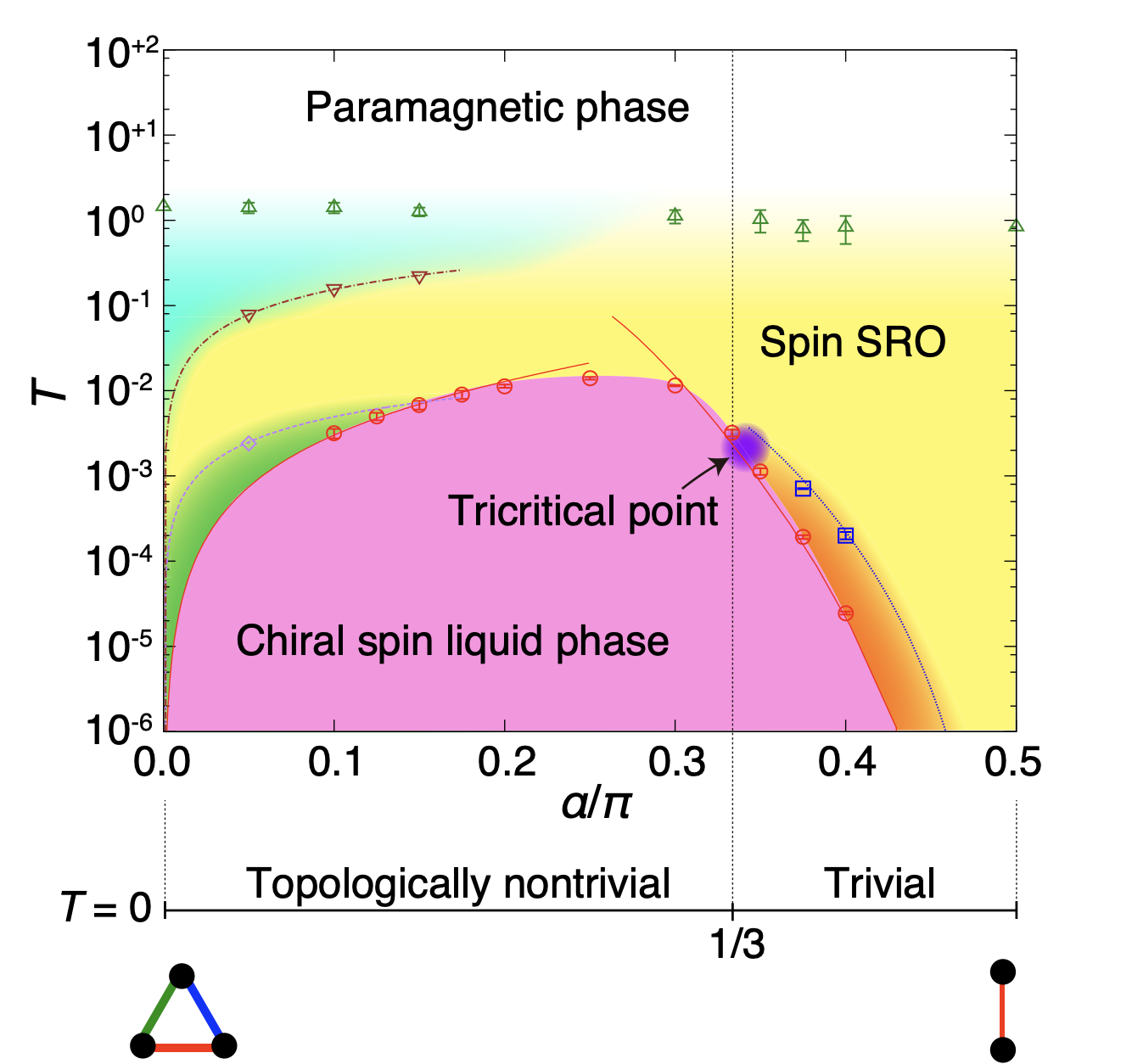
Chiral spin liquids, which break time-reversal symmetry, have garnered interest not only in magnetic materials but also in quantum information, as their elementary excitations may serve as non-Abelian anyons for topological quantum computation.
To elucidate the thermodynamic properties of chiral spin liquids, this study focused on the Kitaev model on a two-dimensional decorated honeycomb lattice, where the ground state is a chiral spin liquid breaking time-reversal symmetry.
The model can realize two phases with distinct topological properties of quasiparticles by varying interaction strength.
Using a quantum Monte Carlo method incorporating Majorana fermions, we analyzed the finite-temperature properties of this model.
The results revealed phase transitions associated with the breaking of time-reversal symmetry at finite temperatures.
Furthermore, we found differences in the order of transitions in phases with different topological properties, indicating the existence of a tricritical point.
This suggests that the topological properties of quasiparticles influence thermodynamic properties.
J. Nasu and Y. Motome: Phys. Rev. Lett. 115, 087203 (2015).
Y. Kato, Y. Kamiya, J. Nasu, Y. Motome: Phys. Rev. B 96, 174409 (2017).
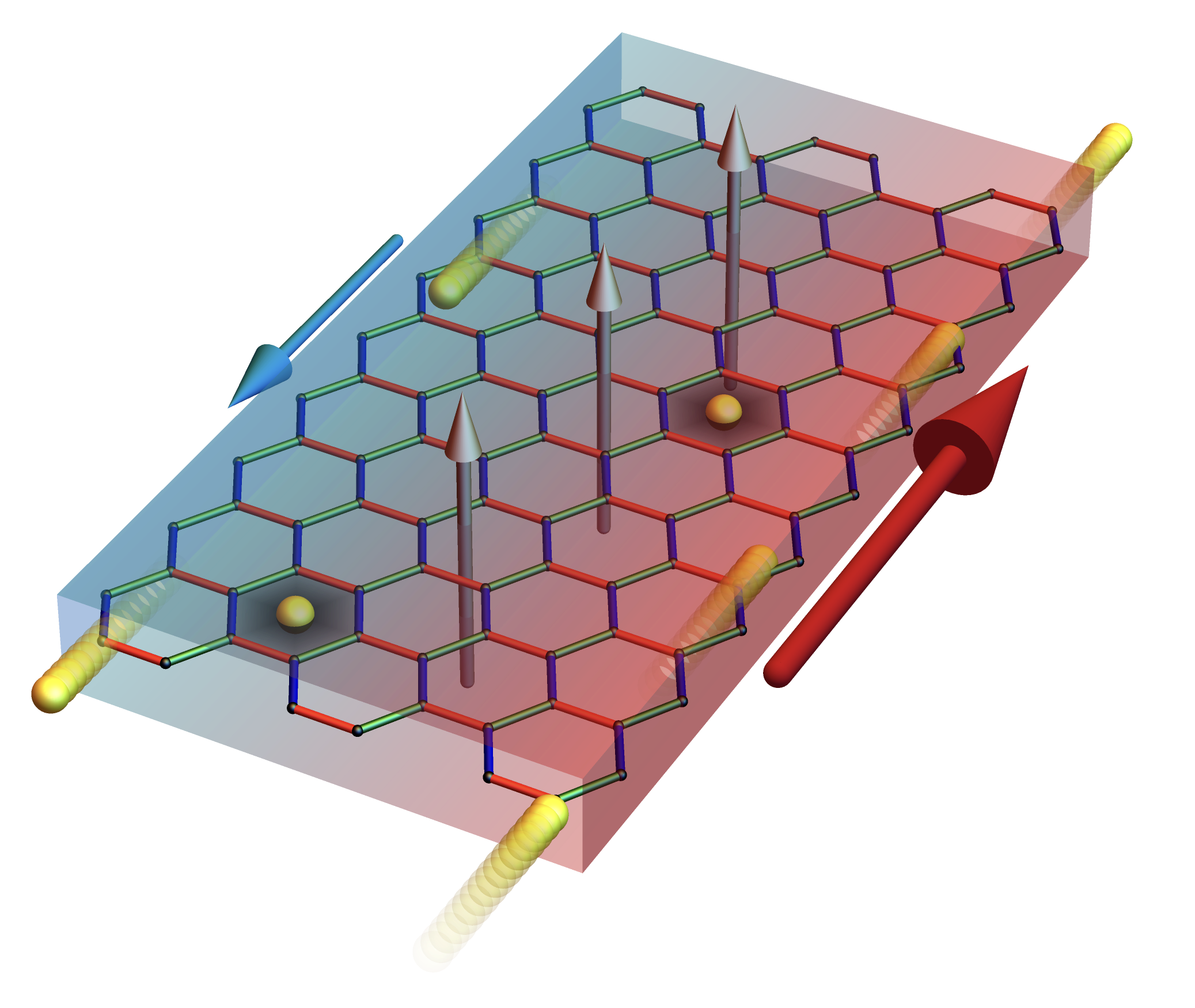
In quantum spin liquids that exhibit no magnetic order down to extremely low temperatures, spin fractionalization is evidenced by experimental measurements such as the asymptotic behavior of specific heat at very low temperatures.
For comparison with these experiments, finite-temperature theoretical calculations are essential, though understanding the properties of quantum spin liquids is challenging even at zero temperature.
This study applied quantum Monte Carlo methods to the Kitaev model, which has a quantum spin liquid as its exact ground state, to investigate its thermal conductivity in detail.
In this model, fractionalization of quantum spins gives rise to emergent Majorana particles that transport heat.
The longitudinal thermal conductivity exhibits a peak at temperatures corresponding to the energy scale of interactions.
Upon introducing a magnetic field, the longitudinal thermal conductivity remains nearly unchanged, while the thermal Hall conductivity becomes finite and is quantized at zero temperature.
We found that this quantity strongly depends on the magnetic field and exhibits nonmonotonic temperature dependence.
These features are precursors of quantum spin liquids at finite temperatures, caused by the emergent Majorana particles within them. Measuring thermal Hall conductivity is expected to experimentally capture the presence of Majorana fermions and their edge states.
J. Nasu, J. Yoshitake, and Y. Motome: Phys. Rev. Lett. 119, 127204 (2017).
Y. Kasahara, T. Ohnishi, Y. Mizukami, O. Tanaka, Sixiao Ma, K. Sugii, N. Kurita, H. Tanaka, J. Nasu, Y.
Motome, T. Shibauchi, and Y. Matsuda: Nature 559, 227 (2018).
T. Yokoi, S. Ma, Y. Kasahara, S. Kasahara, T. Shibauchi, N. Kurita, H. Tanaka, J. Nasu, Y. Motome, C.
Hickey, S. Trebst, and Y. Matsuda: Science 373, 568 (2021).
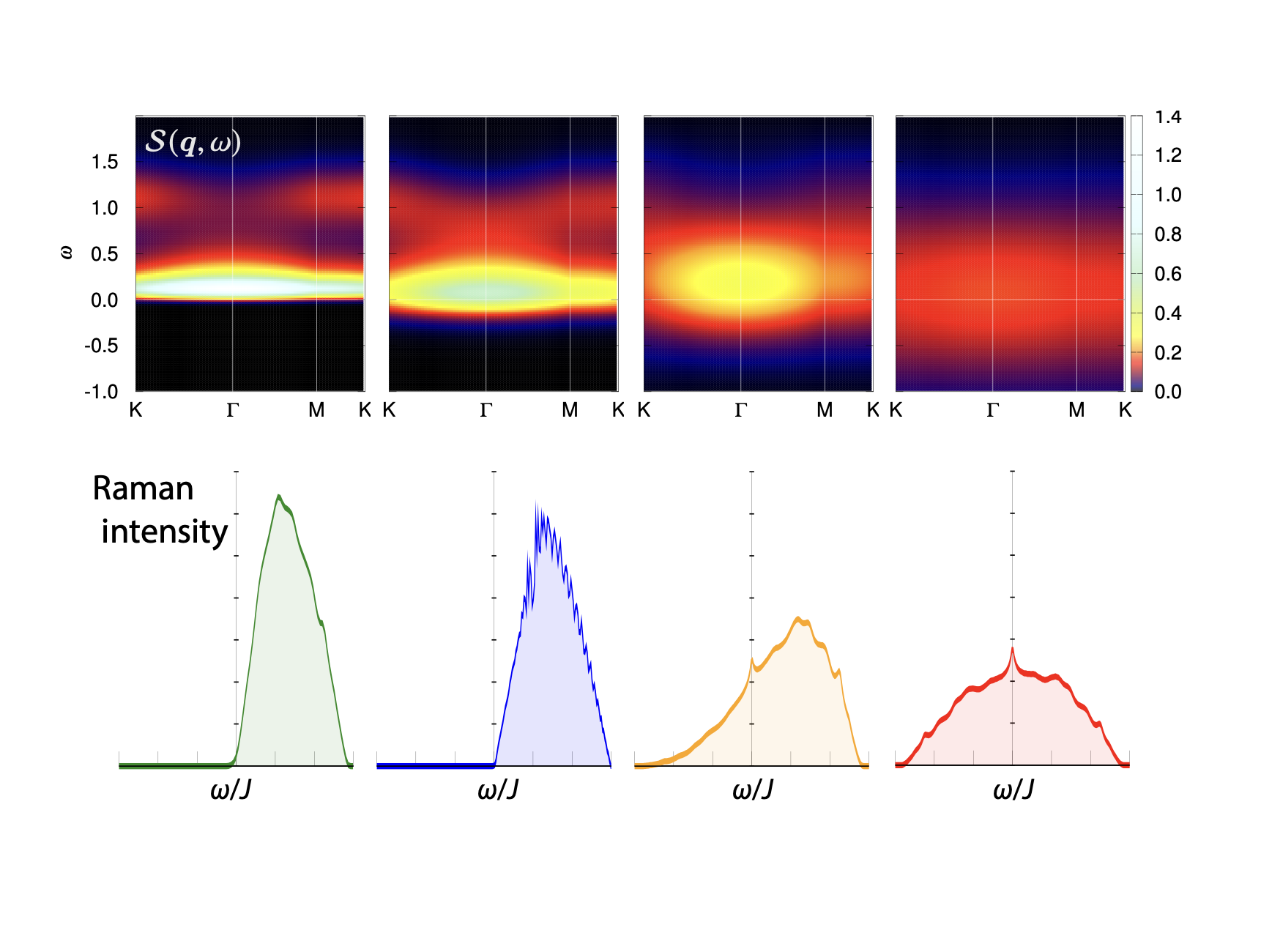
Quantum spin liquids do not have clear order parameters, making them difficult to characterize.
Recent experiments have focused on the fermionic excitations that arise from spin fractionalization, particularly by analyzing how specific heat behaves at extremely low temperatures.
To compare these experimental results, theoretical calculations at finite temperatures are essential.
In this study, we used the Kitaev model, which has a quantum spin liquid as its exact ground state, to compute dynamic magnetic responses at finite temperatures.
Our analysis of Raman spectra revealed a temperature dependence that follows the Fermi distribution function, confirming the fermionic nature of Majorana fermions.
This interpretation closely matched experimental observations. Additionally, other magnetic properties, such as dynamical spin structure factors, magnetic susceptibility, and NMR relaxation rates, also displayed unique temperature-dependent behavior due to spin fractionalization.
J. Nasu, J. Knolle, D. L. Kovrizhin, Y. Motome, R. Moessner: Nature Physics, 12, 912 (2016).
J. Yoshitake, J. Nasu, and Y. Motome: Phys. Rev. Lett. 117, 157203 (2016).
J. Yoshitake, J. Nasu, Y. Kato, and Y. Motome: Phys. Rev. B 96, 024438 (2017).
J. Yoshitake, J. Nasu, and Y. Motome: Phys. Rev. B 96, 064433 (2017).
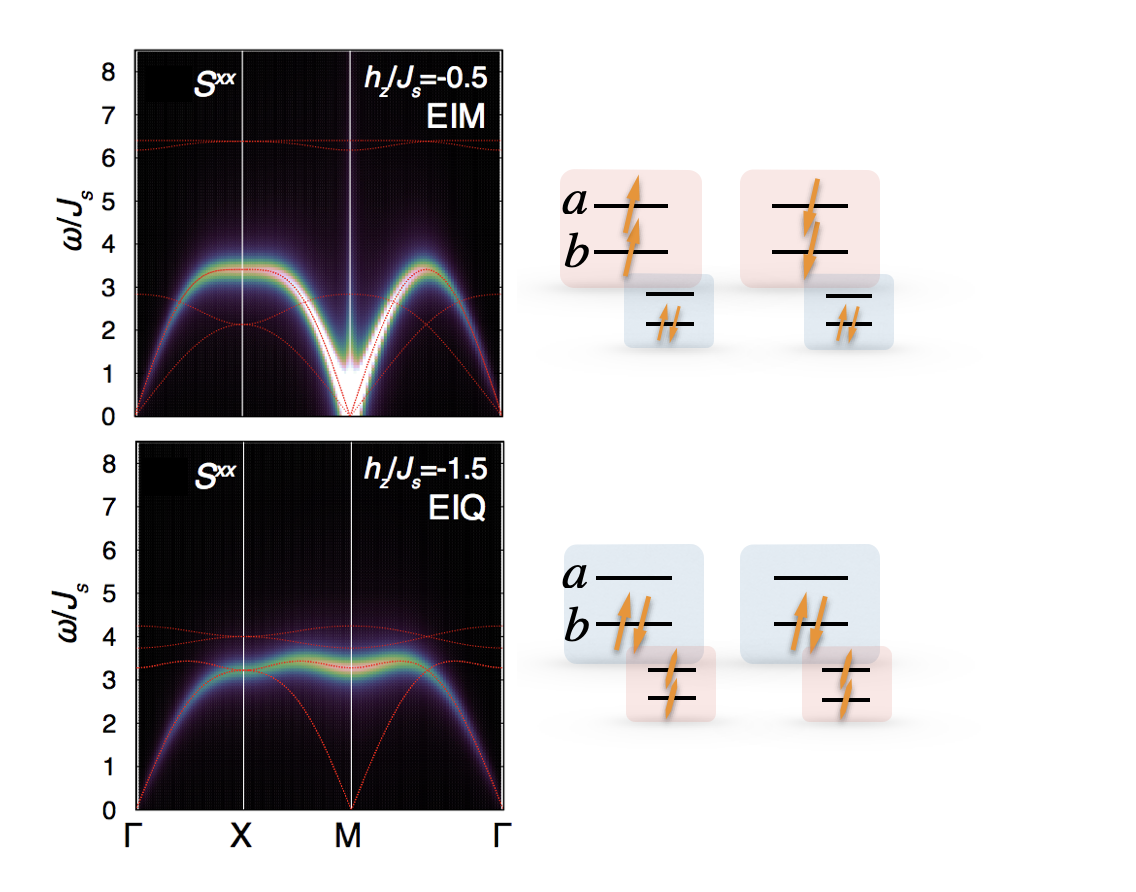
The excitonic insulator state is characterized by spontaneous hybridization between conduction and valence bands, originating from electron-electron interactions. Perovskite cobalt oxides have attracted attention as systems where these interactions are prominent. To elucidate the potential for excitonic condensation due to spin-state degrees of freedom, we analyzed a strongly correlated model based on a two-orbital Hubbard model. The study revealed two types of excitonic condensation states at the boundary between band and Mott insulators. Collective excitations arising from excitonic condensation in these states are expected to be observable in inelastic neutron scattering experiments.
J. Nasu, T. Watanabe, M. Naka, and S. Ishihara: Phys. Rev. B 93, 205136 (2016).
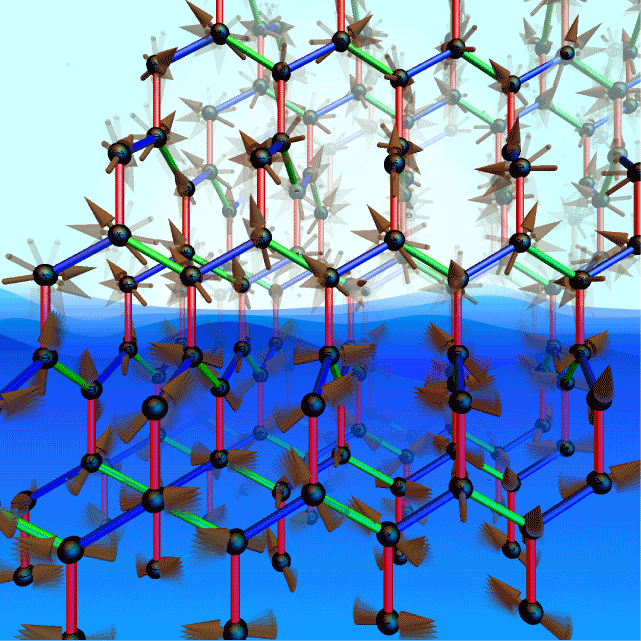
Magnetic materials exhibit a paramagnetic state with disordered spins at high temperatures and transition to a magnetically ordered state with broken symmetry at lower temperatures.
In 1973, P.W. Anderson proposed the concept of a “quantum spin liquid” as a novel quantum state that possesses strong correlations but lacks magnetic order, analogous to the “liquid” phase of matter.
However, due to theoretical challenges, the thermodynamic properties of quantum spin liquids remained largely unexplored.
To address this, we developed a quantum Monte Carlo method based on the Majorana representation for the three-dimensional Kitaev model, which has a quantum spin liquid as its exact ground state.
Our analysis revealed a finite-temperature phase transition from the high-temperature paramagnetic state to the low-temperature quantum spin liquid, characterized by a topological change in the excited state.
These findings challenge the previously assumed adiabatic continuity between paramagnetic and quantum spin liquid states in experimental studies and may have significant implications for quantum computing applications.
J. Nasu, M. Udagawa, and Y. Motome: Phys. Rev. Lett. 113, 197205 (2014).
J. Nasu, M. Udagawa, and Y. Motome: Phys. Rev. B 92, 115122 (2015).
Y. Kamiya, Y. Kato, J. Nasu, and Y. Motome: Phys. Rev. B 92, 100403(R) (2015).
J. Nasu, Y. Kato, J. Yoshitake, Y. Kamiya, Y. Motome: Phys. Rev. Lett. 118, 137203 (2017).
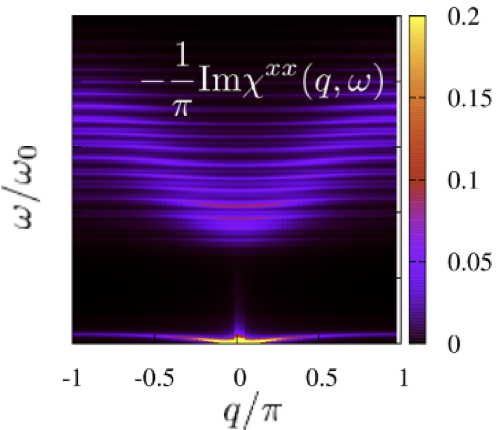
Orbital excitations, elementary excitations from orbital order in strongly correlated electron systems, have been extensively studied experimentally and theoretically.
These excitations are expected to strongly couple with lattice vibrations due to the Jahn-Teller effect.
However, previous theoretical studies have not fully addressed the impact of the Jahn-Teller effect on orbital excitations.
This study extended the spin-wave approximation, combined with mean-field approximation, to analyze the influence of the Jahn-Teller effect regardless of its strength.
The results showed that even under strong Jahn-Teller effects, low-energy orbital excitations accompanied by phonons exist.
J. Nasu and S. Ishihara: Phys. Rev. B 88, 205110 (2013).
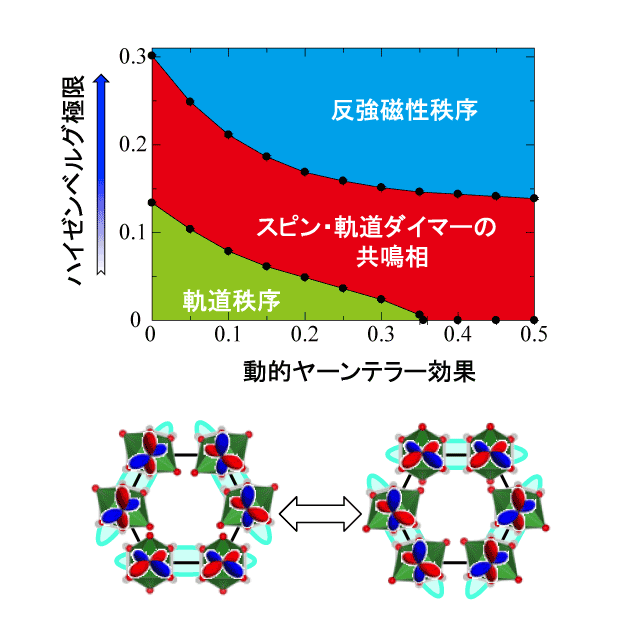
In Mott insulators with orbital degrees of freedom, superexchange interactions, known as Kugel-Khomskii interactions, and the coupling between electronic orbitals and lattice vibrations, known as Jahn-Teller effects, play crucial roles.
These interactions typically stabilize magnetic and orbital orders.
However, in the layered copper oxide Ba3CuSb2O9, no magnetic or orbital order is observed even at extremely low temperatures [A. Nakatsuji et al., Science 336, 559 (2012)].
Focusing on dynamic Jahn-Teller effects, we derived a low-energy effective model incorporating both interactions, using Ba3CuSb2O9 as a reference.
Cluster mean-field analysis revealed a novel quantum state with strong entanglement between orbital, spin, and lattice degrees of freedom, realized between magnetically and orbitally ordered phases.
This disordered state is expected to correspond to the quantum spin liquid state observed in Ba3CuSb2O9.
J. Nasu and S. Ishihara: Phys. Rev. B 88, 094408 (2013).
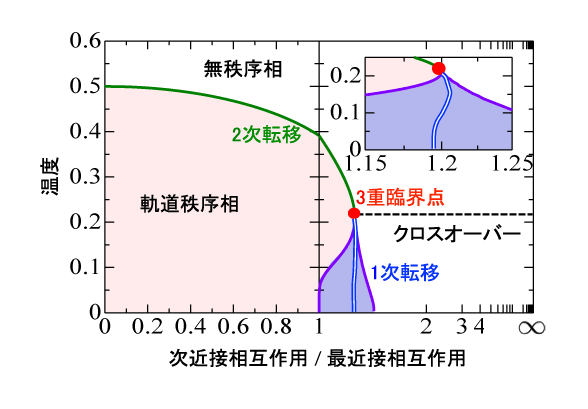
Orbital degrees of freedom introduce spatial anisotropy in electronic charge distributions, significantly influencing the presence or absence of long-range order depending on the lattice's geometric structure.
To investigate the novel orbital states arising from these effects, we introduced a checkerboard compass model featuring both orbital anisotropy and geometric frustration by considering doubly degenerate orbital degrees of freedom on a checkerboard lattice.
Using multiple analytical methods, including spin-wave approximation, mean-field approximation, one-dimensional exact solutions, quantum Monte Carlo simulations, and exact diagonalization, we analyzed this model.
Near the frustration point, where interactions along two bond directions compete, reentrant behavior of orbital order was observed, attributed to an "order by disorder" mechanism induced by thermal and quantum fluctuations.
Additionally, orbital anisotropy was found to give rise to a tricritical point and enhance low-energy one-dimensional excitations in orbital excitation spectra.
J. Nasu, S. Todo, and S. Ishihara: Phys. Rev. B 85, 205141 (2012).
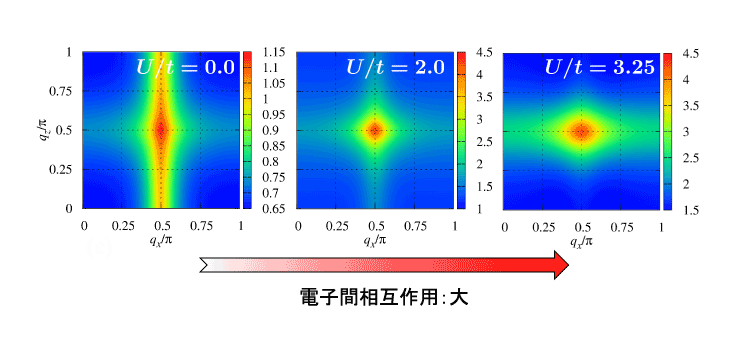
Orbital degrees of freedom, representing the spatial anisotropy of electronic distributions, strongly influence the directional dependence of orbital interactions.
In the strongly correlated regime, this anisotropy induces frustration, leading to nontrivial ground states.
Complementing this perspective, we analyzed the weak correlation regime to investigate how orbital frustration affects orbital states.
Introducing a Hubbard model on a square lattice that reduces to a compass model in the strongly correlated limit, we analyzed it using random phase approximation (RPA) and self-consistent RPA.
In the absence of electron-electron interactions, orbital susceptibility exhibited a one-dimensional divergence in directions different from those predicted in the strong correlation regime.
However, in the intermediate interaction region, this one-dimensional divergence disappeared, and susceptibility anisotropy diminished.
This behavior was attributed to suppression of nesting-driven susceptibility divergence by orbital anisotropy.
These features are not exclusive to the compass model but are also realized in orbital models describing the anisotropy of eg orbitals.
J. Nasu, and S. Ishihara: Europhys. Lett. 97, 27002 (2012).